The WiscAr Laboratory collaborates with researchers and institutions from around the world. While we work on numerous small research projects, the lab also focuses on large scale innovative studies that use geochronology, geochemistry, and petrology to better understand Earth’s history.
Here are some of the major research projects the WiscAr team are currently working on:
Ice forcing in arc magmatic plumbing systems (IF-AMPS)
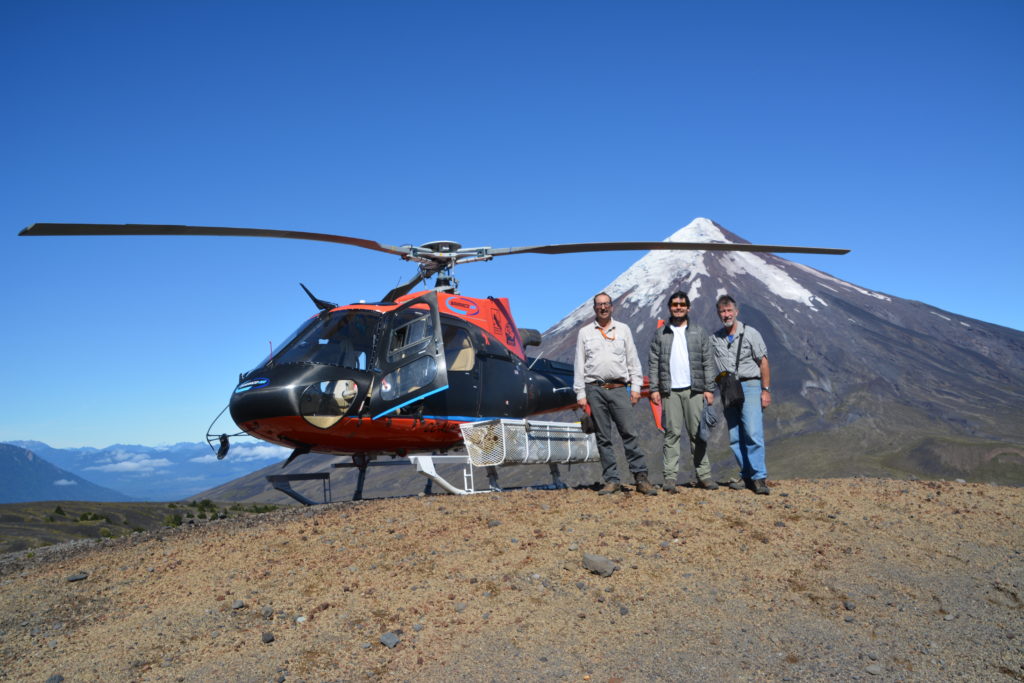
From left to right: Brad, Pablo, and Brent Alloway (senior volcanologist) conducting fieldwork on Osorno volcano
A question at the frontier of Earth science is: how do changes in the climate system on our planet’s surface interact with magma reservoirs housed within its interior? We will conduct a novel blend of field observations, lab measurements, and numerical model simulations in an integrated study of links between changes in glaciers and topography, and the behavior of several active volcanoes in Chile during the last 50,000 years. These volcanoes were partly covered by the 3,000 foot thick Patagonian ice sheet until it melted rapidly beginning 18,000 years ago. This natural laboratory offers unparalleled means to investigate how the rapid loss of ice impacted the composition and rates of eruptions from these volcanoes. This project will provide career-building experience for several PhD students. A volcano & ice Summer program will engage technical school students from underrepresented groups in the US and Chile in field- and lab-based experiences, including training in drone technology for data collection and geologic mapping. Our collaborations with Chilean scientists and educators aim to: (1) enhance knowledge of the growth rates and eruptive histories of several of the most dangerous volcanoes in South America, thereby improving hazard assessment, (2) generate new climate proxy data critical to calibrating our numerical model of ice sheet retreat, and (3) train students from the communities living near these volcanoes.
Utilizing new and existing geochronologic, geochemical, glacial and erosion/deposition observations within the Andean Southern Volcanic Zone, we aim to couple a suite of numerical models to test and refine three hypotheses: (1) Over short timescales (<100,000 year), the composition, volume, and timing of eruptions are strongly influenced by climate-driven changes in surface loading. These short-term responses modulate the long-term (>100,000 year) average eruptive characteristics, which are governed by mantle melt flux, (2) Crustal stress changes associated with the local onset of rapid deglaciation and erosion at 18,000 years ago promoted eruptions by enhancing volatile exsolution that in turn pressurized stored magma and propelled dike propagation to the surface, and (3) Responses to rapid unloading will vary among volcanoes, reflecting contrasts in the composition, volatile contents, and compressibility of stored magma, as well as the rate at which crustal reservoirs are recharged from depth. This variability can be exploited to reveal fundamental controls on the sensitivity of glaciated arcs to the climate system. To investigate these hypotheses, we will pursue four objectives: (1) Generate high-resolution records of cone growth, eruptive behavior, and geochemical evolution of six volcanoes during the last ~50,000 years spanning 250 km along the subduction zone, (2) Build new records of ice retreat, and landscape evolution owing to the erosion, transport, and deposition of sediment adjacent to the six volcanoes, (3) Use the observed chemical and physical patterns in the volcanic, climatic, and topographic records to constrain crustal loading through time, and explore the effects of this forcing in numerical models, and (4) Integrate findings to contextualize processes in continental settings, and provide a framework for examining the sensitivity of arc volcanism to external forcing elsewhere and across a spectrum of climate states throughout Earth history.
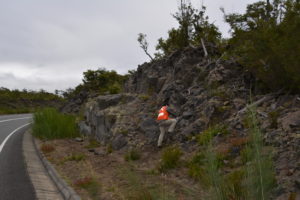
Pablo collecting samples from the 1835 eruption at Osorno volcano. This eruption was glimpsed by Charles Darwin during his second voyage on the Beagle
NSFGEO-NERC: Foundation for the next generation of paleoceanographic and biogeochemical studies: Developing a new Lower Cretaceous time scale
The Cretaceous was a time of global warming during which atmospheric carbon dioxide levels surpassed 800 ppm, similar to that predicted for 2100 by “business-as-usual” emission scenarios. Cretaceous rocks record high sea levels, high biological productivity, massive volcanism, and major perturbations of the carbon cycle during several ocean anoxic events (OAEs). Understanding these phenomena can provide deep-time analogs for future greenhouse scenarios. Marine sediments that record these crises are globally widespread, but weaving these records into a common temporal framework is essential if they are to reveal the drivers. This project aims to generate a new temporal framework spanning 125 to 93 million years ago during which several major OAEs occurred. This will permit examination of repeated deteriorations of marine ecosystems across the Pacific, Atlantic, and Mediterranean oceans. Novel outreach, international collaboration, and training of future earth science leaders feature prominently. We will bring deep-time science to the public via presence at the Sturgis Motorcycle Rally.
The favored hypothesis for OAEs involves volcanic initiation leading to a cascade of processes amplifying global marine production; key factors are the nature of volcanism and the source of increased nutrients. Yet geographic differences in proxy records (C and Os isotopes) indicate additional complexities, such as sea level and ocean circulation. Our goals are to establish: (1) A new time scale for global geochemical and paleobiologic datasets; (2) Chemostratigraphic correlation of the new time scale to European sections using C and Os isotope stratigraphy, and (3) A new global time scale for improved understanding of major biogeochemical perturbations. We will: (i) Determine radioisotopic ages of rhyolitic tuffs in sediments of Japan, (ii) Integrate these new ages with new Os-, and C-isotope chemostratigraphy in Europe, (iii) Compile global geochemical proxy data for OAE1a within a common temporal and stratigraphic framework, and analyze trends and patterns from the Pacific to Europe, (iv) Evaluate the volcanic versus climatic/orbital hypotheses for OAE initiation, and (v) Explore the significance of geography in the timing and magnitude of geochemical signals.
Green River Early Eocene Climate Observatory (GREECO)
Deep-time geologic records of unusually warm climate are vital for ascertaining how the Earth system will respond to rising temperatures in the future. Recent studies have shown that the Early Eocene Climatic Optimum (EECO), between ~50-53 Ma, may be particularly instructive because it embodied a number of relatively abrupt warming episodes. Two factors currently impede our understanding of these hyperthermal events however. First, the early Eocene timescale is only sparsely populated by radioisotopic dates and therefore subject to relatively large uncertainties. Second, the elevated pCO2 levels associated with hyperthermals caused oceanic pH to decrease and the carbonate compensation depth (CCD) to rise. Oceanic records of hyperthermal events are therefore typically condensed and associated with evidence for carbonate dissolution. Alkaline lake strata offer an alternative class of climate archives, that are not subject to the same limitations. The lacustrine Green River Formation and equivalent alluvial deposits offer an especially important opportunity to complement the marine record of the EECO. These nonmarine strata contain a uniquely rich, expanded stratigraphic record of climate change, as well as numerous tephras. In addition to advancing our understanding of EECO climate, these deposits offer a rare opportunity to intercalibrate early Eocene radioisotopic, magnetostratigraphic, and astrochronological timescales.
We are addressing three critical questions regarding Eocene climate through an integrated, multidisciplinary investigation that focuses on one of the world’s richest and best studied systems of ancient lake strata — the Eocene Green River Formation (GRF) in the western U.S. Our team will investigate key interrelated hypotheses: 1. How did the timing of terrestrial warming compare to that inferred from the marine record, and to predicted patterns of astronomical forcing? Recent advances in radioisotopic dating can be used to directly test the relationship of warming events to orbital forcing, calibrate dynamic gravitational models of the solar system back to ~50 Ma (Laskar, 2011), and improve the accuracy of the early Eocene timescale. 2. How did temperatures, seasonality, hydrologic cycling, and weathering on land evolve during the EECO? We hypothesize that warming was amplified on continents relative to the oceans and was accompanied by decreased seasonality, while precipitation seasonality and rainfall intensity increased. Precession-scale variability of the hydrologic cycle was generally linked to wintertime Pacific storms, while eccentricity-paced hyperthermal events were expressed as episodic increases in summer precipitation from the North American monsoon. 3. How did the complex tectonic and magmatic evolution of western North America impact the preservation of EECO climate signals?
We are testing the hypothesis that repeated, stepwise drainage reorganizations within the Laramide foreland modified the hydrologic balance and sedimentary facies of Eocene Lake Gosiute and its response to orbital forcing.
EarthCube Integration: Geochronology Frontier at the Laboratory-Cyberinformatics Interface
This grant will support a partnership between geochronologists who have built and run laboratory facilities that are designed to measure the ages of rocks using radioisotopic and astronomical methods, geoscientists who are building synthetic databases that depend critically on accurate and precise ages of rocks in order to test hypotheses in the Earth and life sciences, and computer scientists who are building infrastructure components that are now being used broadly in education and research. The aim is to address a ‘grand challenge’ in the Earth sciences: to develop a fully integrated four-dimensional digital Earth so that we may fully understand dynamic Earth system evolution through time. To meet this goal we plan to develop: (1) a robust cyberinfrastructure to manage and expose data produced by multiple distributed geochronology laboratory facilities around the USA, (2) a digital mechanism to enable scientists of all types to readily discover and use geochronologic data, while at the same time keeping age estimates closely connected to geochronology lab expertise and underlying, laboratory-specific data, (3) protocols and workflows that pass geochronological data and metadata from labs to synthetic geological and paleobiological databases and data repositories, and (4) software that can harness this new geochronological infrastructure and leverage it in order to generate age models for broad swaths of rocks and thereby enable the correlation of Earth system records across a range of nested spatial and temporal scales. Institutions formally collaborating in these efforts include: University of Wisconsin-Madison, University of Arizona, Boise State University, New Mexico Institute of Mining and Technology, and University of Minnesota.
Geochronological data are central to our understanding of Earth’s past and future. This collaboration between geo- and computer scientists will: (1) create cyberinfrastructure that better leverages existing and new laboratory-generated geochronologic data, and (2) integrate this infrastructure with with synthetic databases including: Paleo Biology Data Base (https://paleobiodb.org), Neotoma Data Base (https://www.neotomadb.org), Macrostrat (https://macrostrat.org), as well as the Integrated Earth Data Alliance (IEDA) Geochron (https://www.geochron.org/) data repository using a standard that can be widely applied by others. Our approach is unique in that it involves the parallel efforts of both the producers and consumers of geochronologic data as well as technical staff who have a working knowledge of geologic and biologic databases. Our team is also well positioned to broadly serve geochronology because it engages three different geochemical/radioisotopic systems that address different geological problems and time scales. One of the overarching science goals of EarthCube is to characterize the key processes, interactions and feedbacks operating at and across different temporal and spatial scales and biological, chemical, mechanical, and physical domains. These modest, but concrete, steps will allow us to develop templates for distributed, laboratory cyberinfrastructure and geochronologically grounded models that can be adapted and used across Earth science communities. The aim is to share best practices and move Earth scientists towards an open, frictionless transfer of data and knowledge. The impacts of this project extend beyond our laboratories and collaborations and include: (1) Three young geoscientists (1 PhD student and 2 Postdoctoral scholars) will emerge with the cyberinformatics experience to become next generation faculty/research leaders, (2) a set of standards for distributed laboratory server operations will be created and implemented, forming the foundation for establishing a global network of lab-derived geochronological data, and (3) the GeochronAPI deployed across this network will provide a mechanism by which to integrate and synthesize geochronological data into independently developed applications, including geodiscovery-oriented Flyover Country (https://fc.umn.edu). Through workshops we will engage a wide spectrum of geochronologists to converge on an open standard for the GeochronAPI system, and our work with the synthetic databases highlighted above will allow us to engage a large community spanning bio- and geoscience. All of our software, both lab-centric and external facing, will be made accessible in public GitHub repos and we will encourage open, creative development.